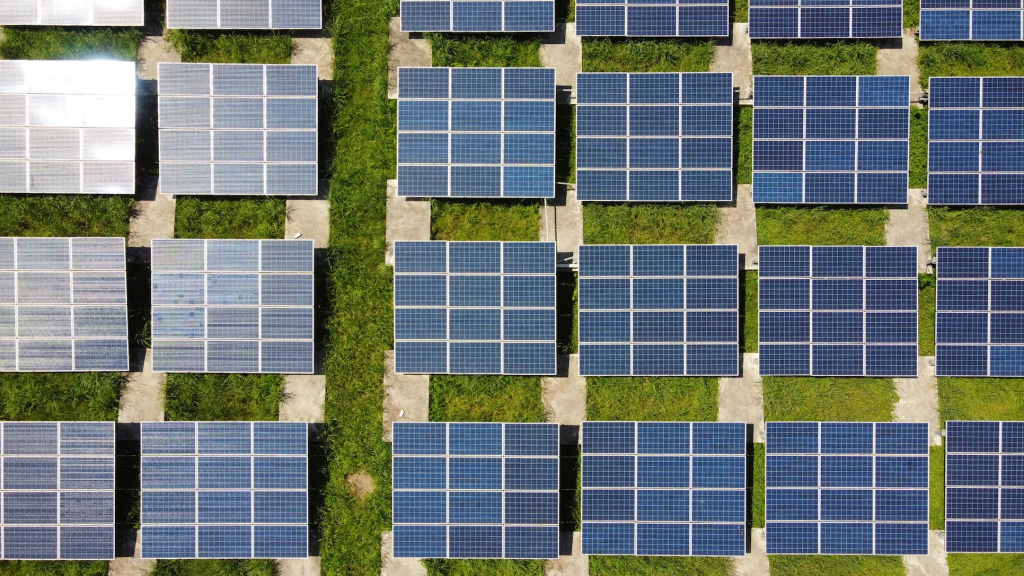
Starting in the mid 2000s, I often introduced students in my science classes to sustainable energy by referring to Desertec, a non-profit foundation that focuses on the production of renewable energy in the desert regions of north Africa. Initially, the project relied on concentrated solar energy to produce thermal energy = heat that could be stored in salt. This thermal energy was then to be used to produce electricity. The aim was to create a global renewable energy plan based on the concept of harnessing sustainable powers, from sites in Africa and the Middle East where renewable sources of energy are more abundant, and transferring it through high-voltage direct current transmission lines to consumption centers, in Europe.
I lost interest when this proved to be uneconomic, and thus unworkable. The foundation’s next idea was to focus on meeting domestic demand. This also failed because of transportation and cost-inefficiency issues. The initiative was revived in 2020 with a focus on green hydrogen, catering to both domestic demand and exports to foreign markets. This failed to spark my interest, because I regard the transport of a product (in this case, hydrogen) to be more problematic than transporting electrical energy.
One of the main reasons why Desertec faces problems, is that since the early 2000s, solar cells and panels have become increasingly inexpensive, and provide some of the cheapest sources of electricity on the planet. They can be effectively used in most places where people live.
The solar cell was invented when Russell Shoemaker Ohl (1898 – 1987), a researcher at Bell Labs, noticed in 1940 that a cracked silicon sample produced a current when exposed to light. He patented the modern solar cell, described in US Patent 2402662, “Light sensitive device”, filed in 1941.
Martin Green (1948- ), was born in Brisbane, Australia, where he also received a B.Sc. degree from the University of Queensland, before and completed his PhD at McMaster University in Hamilton, Ontario, Canada, where he specialised in solar energy. In 1974. he returned to Australia, where he worked at the University of New South Wales. In 1975, he started a photovoltaic research group, using unwanted scrounged equipment.
Green and his students first worked to increase the voltage of solar cells. When this came up to a satisfactory level, focus was changed to quality improvement and energy efficiency. By 1989 the lab had built solar panels capable of running at 20% efficiency. In 2015, they achieved a 40.6% conversion rate using focused light reflected off a mirror.
Shi Zhengrong (1963 – ) was born in Yangzhong, Jiangsu, China. He completed his undergraduate studies at Changchun University of Science and Technology, and obtained his Master’s degree from the Shanghai Institute of Optics and Fine Mechanics, at the Chinese Academy of Sciences. Afterwards, Shi obtained a Ph.D. in solar power technology at the University of New South Wales. School of Photovoltaic and Renewable Energy Engineering. In 2001, Shi returned to China and set up Suntech Power in Wuxi with $6m in start-up funding from the municipal government.
Suntech made inexpensive, conventional PV solar panels with 17% efficiency. SunTech increased its production capacity from 60 megawatts (MW) to 1 gigawatt in 2009. This was possible because Germany, and other mainly European Union (EU) countries passed legislation encouraging solar power. This resulted in a massive global demand. By 2012, the world market faced excess solar panel supplies, which reduced prices to below cost. After an investment scandal, SunTech was bankrupt by 2013-03.
Other companies ensured continued production of low cost, highly efficient solar panels. The International Energy Agency reports that solar is providing the cheapest energy the world has ever seen.
The problem with solar energy is that it is available only half of the time. However, when that half occurs is largely dependent on latitude. Many producers of solar cells set 60°, as a northern (and southern) limit for their effective use.
Location | Vangshylla | New Westminster | Oakland |
Latitude | 63° 17′ 0″ N | 49° 12′ 25″ N | 37° 48′ 16″ N |
PV Out | 853.0 | 1235.2 | 1728.5 |
DNI | 769.3 | 1336.4 | 2146.9 |
GTI optimal | 992.9 | 1474.9 | 2117.1 |
Tilt opt | 43 | 37 | 33 |
Summer solstice sunrise | 02:48:50 | 05:05:48 | 05:46:58 |
Summer solstice sunset | 23:45:58 | 21:21:08 | 20:34:52 |
Summer solstice daylight | 20:57:08 | 16:15:20 | 14:47:54 |
Winter solstice sunrise | 10:03:51 | 08:03:28 | 07:20:30 |
Winter solstice sunset | 14:53:15 | 16:16:01 | 16:53:53 |
Winter solstice daylight | 04:19:54 | 08:12:33 | 09:23:33 |
PV out can be regarded as the photovoltaic power potential; that is, the relative potential of a location to produce electricity from a solar panel. In the table above, Oakland has twice the capability of Vangshylla, while New Westminster has an intermediate position.
DNI = Direct Normal Irradiation, and is measured in kWh/m2 per year. Here, Oakland can produce three times as much electricity as Vangshylla. GTI = Global tilted irradiation at optimum angle. By setting solar cells at an optimal angle, the amount of electricity produced can be enhanced, considerably in high latitudes. However, to make matters worse, high latitude locations have most of their production in the summer months (when it is needed the least), with very little production in the winter (when it is needed most). At Vangshylla the length of the day varies by 16h:37m:14s. In Oakland, that difference is only 05h:24m:21s.
Note: Most of the content was written on or before 2021-04-25. It was updated immediately prior to publication.
My life approximately spans the history of PV as outlined here and it has been quite amazing to watch the development over this time.