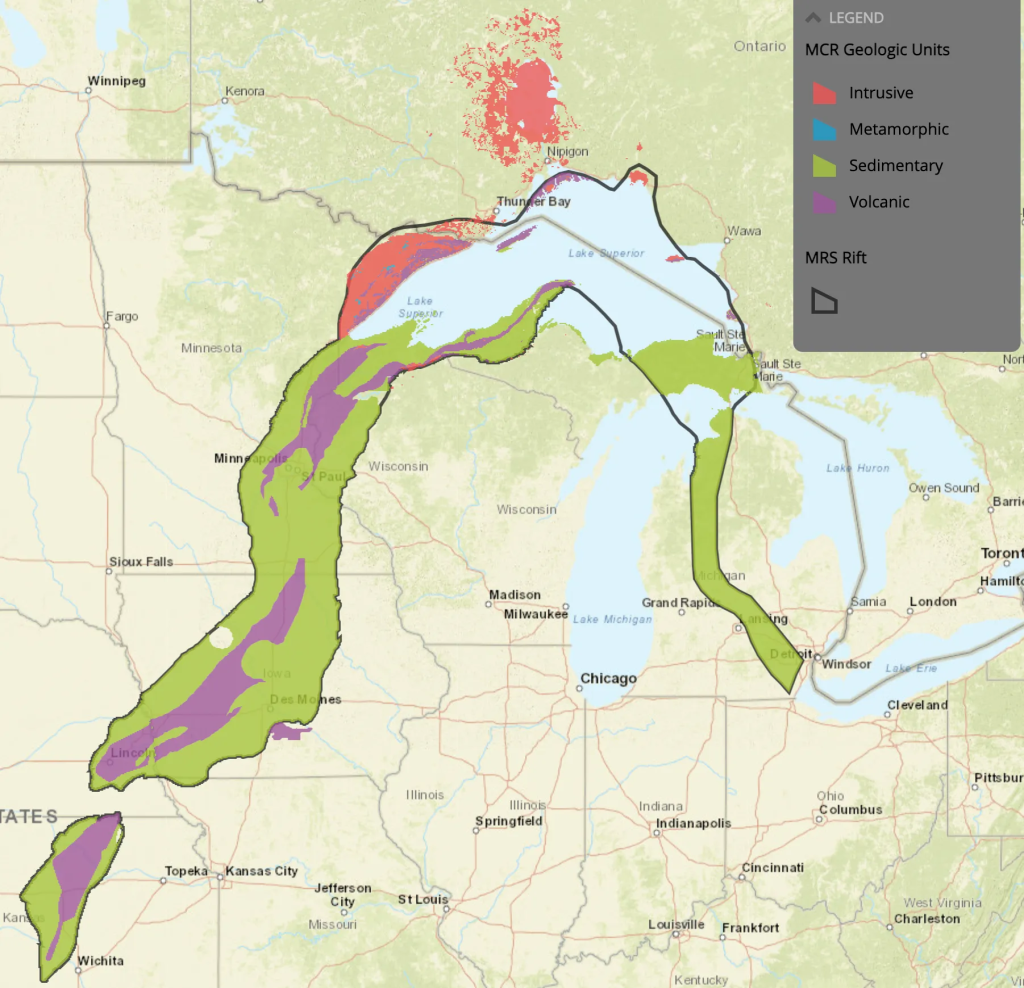
Hydrogen is an important element in a number of compounds, including water (H20), methane (CH4) and ammonia (NH3). In my opinion it is not an effective energy carrier. The accessible energy is stored in electrons.
One hypothetical question about hydrogen is to ask if pipelines should be built to move H2 to other places, or if it is better to move electrons using high-voltage direct current ( HVDC) transmission? It is hypothetical because no energy analyst without a vested interest in pipelines, would bother to ask the question. They know the answer is HVDC.
One avoids a lot of confusion, if the starting point for understanding energy transmission is exergy. Two (slightly modified) paragraphs from Wikipedia can provide a foundation, even if it takes time for one’s brain to understand the concept: Exergy, often referred to as available energy or useful work potential, is a fundamental concept in the field of thermodynamics and engineering. It plays a crucial role in understanding and quantifying the quality of energy within a system and its potential to perform useful work. Exergy analysis has widespread applications in various fields, including energy engineering, environmental science and industrial processes.
From a scientific and engineering perspective, second-law [of thermodynamics] based exergy analysis is valuable because it provides a number of benefits over energy analysis alone. These benefits include the basis for determining energy quality (or exergy content), enhancing the understanding of fundamental physical phenomena, and improving design, performance evaluation and optimization efforts. In thermodynamics, the exergy of a system is the maximum useful work that can be produced as the system is brought into equilibrium with its environment by an ideal process. The specification of an ‘ideal process’ allows the determination of ‘maximum work’ production. From a conceptual perspective, exergy is the ideal potential of a system to do work or cause a change as it achieves equilibrium with its environment. Exergy is also known as ‘availability’. Exergy is non-zero when there is dis-equilibrium between the system and its environment, and exergy is zero when equilibrium is established (the state of maximum entropy for the system plus its environment).
With that out of the way, in the course of this post, one will look at four academic papers. The two earliest academic papers considered here, got numerous facts wrong, the other two got some things right. The first two were: Relative costs of transporting electrical and chemical energy (2018) by Saadi et al and Cost of long-distance energy transmission by different carriers by DeSantis et al (2021).
One assumption, in both papers, is the existence of a large source of green hydrogen molecules available at a single location suitable for putting into a 1 000 km pipeline. Hydrogen plants, close to existing pipelines, don’t use green hydrogen but grey hydrogen based on a centralized natural gas steam reformation system. For a short explanation of hydrogen’s colours scroll down to the end of this post.
The papers assume green hydrogen is inexpensive to manufacture: $1 per kilogram in the 2018 study; $2-$4 per kilogram per the 2021 study. Yet, green hydrogen uses 50 – 60 kWh of firmed electricity per kilogram to manufacture. Firmed electricity costs about $0.10 per kWh, unless one is using massive legacy hydroelectricity facilities found in Norway, Quebec and British Columbia. Here $0.5-$0.06 per kWh could be found. Firmed is a term used to describe energy that can be constantly provided at a needed rate and at a fixed cost.
A better estimate of the manufacturing cost of hydrogen is $5 to $10 per kilogram.
There are also incorrect assumptions about the capacity of HVDC transmission lines, underestimating capacity. In 2019, a Chinese 1 .1 MV = 1 100 kV link was completed. It traverses a distance exceeding 3.3 Mm = 3 300 km = 2 100 miles with a power capacity of 12 GW.
At the end of every line (pipe, as well as transmission), energy has to be distributed onward. Electricity is part of a high efficiency (read: cheap) distribution grid. Hydrogen is expensive to distribute, often relying on trucks with specially made pressurized tanks. While gray hydrogen costs $1-$2 to manufacture, it currently sells for €15 to €25/ kg to consumers in Europe and $30 to consumers in California. Much of this difference in price is due to distribution costs.
Both papers ignore that hydrogen as an energy carrier has to be used. Molecules are less efficient than electrons. Fuel cells offer only about 50% efficient in turning H2 into electricity, while electricity stored in batteries is much more efficient.
Burning hydrogen for heat under 200° C = 392 ° F competes directly with heat pumps, which are three times as efficient on average, and in industrial settings usually even more efficient. This means there is no need for H2 for residential or commercial heating.
The vast majority of industrial processes working above 200°C are electrifiable, typically with efficiency gains over using burnable fuels. The hydrogen pipeline studies ignore this inconvenient truth.
A Norwegian DNV (previously Den norske veritas) study, Specification of a European Offshore Hydrogen Backbone (2023) produced a report for The European Association of pipelines. It is slightly more realistic than the 2018 and 2021 studies, but still contains major flaws. It contends that green hydrogen can be delivered to the end of a transmission line for €3.21 per kilogram in 2050, and that this is cheaper than the transmission of electrons. It uses more expensive HVAC transmission rather than HVDC transmission, and exaggerates these transmission losses compared to hydrogen pipeline efficiencies. They also overstate transmission line operating costs, despite the fact that HVDC has no moving parts while pipelines have lots of moving parts, such as pumps.
Despite all of the unrealistic benefits given to H2, it’s still ten times more expensive to transport than liquid natural gas (LNG). Michael Barnard commenting on the report in CleanTechnica, contends that the report “was structured to meet the need of the clients to pretend that manufacturing molecules of hydrogen offshore at wind farms and then constructing pipelines all the way to major demand centers was the most cost effective model, and the DNV analysts contorted numbers and the space time continuum until the client’s needs were satisfied.”
Hydrogen pipelines vs. HVDC lines: Should we transfer green molecules or electrons? (2023) by the Oxford Institute for Energy Studies is better. It starts with the same amount of electricity at the beginning of the pipeline and HVDC = 9.6 GWh. They then apply most electrolysis facility energy losses before putting hydrogen into the pipeline, and apply the much smaller efficiency losses to electricity before it gets into transmission.
They calculate the energy delivered at the end of the pipeline to be 1.15 – 5.71 GWh , with an average value of about 3.4 GWh or a 34% delivery rate. At the end of the transmission line, there is 7.872 – 8.832 GWh, with an average value of 8.35 GWh or 87% delivery rate.
Yes, there is considerably more energy at the end of the transmission line. The hydrogen pathway delivers only 40% of the energy compared to the electron pathway. In economic terms, that means that energy from H2 will cost 2.5 times what it costs using electricity.
Much of the rest of the report can be filed under magic/ apologetics for the H2 industry, with unsupported contentions, and unrealistic costs: too little for hydrogen, but too big for electricity.
Other weaknesses in the report include failure to explain how one can get firmed electricity in sufficient quantities to an offshore electrolysis facility economically, or to take into account construction costs. In addition, electrolysis is simplified, missing several components such as a hydrogen dehumidifier.
At the other end of the pipeline, exergy is not taken into account. They treat the energy delivered as molecules and the energy delivered as electrons as being equal. Fuel cells are only 50% efficient at turning hydrogen into electricity. This means that using hydrogen requiring 5 times as much electricity, in contrast to using electricity directly or with intermediate storage using batteries or capacitors. To produce heat < 200° C, 7.5 times as much electricity is needed, For heat > 200° C, an electric option is almost always more efficient.
I will not state that there is no market for hydrogen for energy, and look specifically at the aviation industry. However, there are no hydrogen pipelines planned (that I can find), although some designs exist. There are many HVDC transmission lines approved, under construction and in operation.
There is a market for H2 as a feedstock/ commodity. In the United States the most economical way of producing it is to pump water down to hot iron-rich rock to extract white hydrogen in the Mid-Continental Rift System (MRS) = Keweenawan Rift. This can be done at an industrial scale. The rift is about 2 000 km = 1 200 miles long, in the center of the North American continent but south-central part of the North American plate. It formed when the continent’s core = the North American craton, began to split apart during the Mesoproterozoic era, about 1.1 billion years ago. When the rift failed, it left behind thick layers of igneous rock that are exposed in its northern reaches, but buried beneath later sedimentary formations along most of its western and eastern arms. This rift is also an important location for metals, but that is a topic for another day.
Conclusion: It makes no economic sense to put electrons into electrolyzers to make H2, then to put that H2 into pipelines. Hydrogen is an ineffective carrier of energy. No hydrogen pipelines will be built to transport energy. HVDC transmission lines will carry electrons everywhere. If H2 is needed as a feedstock, it will be manufactured at the point of use, to avoid transportation costs.
Notes: The various colours of hydrogen are a shorthand way of discussing hydrogen production characteristics. These were listed in an earlier weblog post, although white hydrogen was missing. In summary these are: black/ brown = from coal; grey = from methane/ natural gas; green = from surplus renewable energy; yellow = from solar (my personal usage, others use orange); orange = from mixed sources, including up to 50% non-renewables; red = from mixed sources where non-renewables exceed 50%. pink = from nuclear energy; turquoise (in general use) = purple (my personal usage) = more climate friendly blue hydrogen, such as methane pyrolysis. White (or gold) = hydrogen made by natural processes.